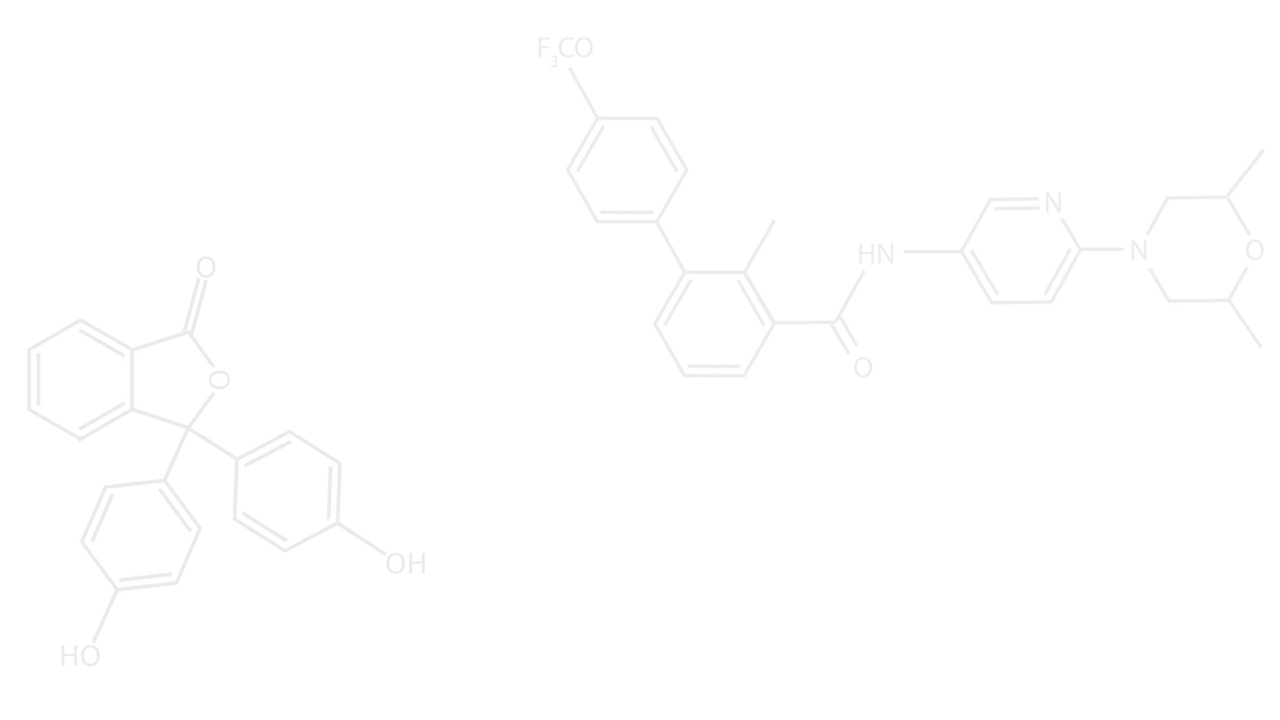
COMETS
Comets are particularly interesting celestial objects, not only since they are so beautiful, but also given their scientific value. Comets are typically kilometer sized. The biggest comets we've seen have radii of dozens of kilometers, however these are extremely rare. The main difference between small asteroids and comets is that comets contain volatiles adequately close to the surface, and when they get within a sufficiently close distance to the Sun, these volatiles receive enough heat, leading to their sublimation (transition from solid to gas) and breaking away from the comet. We refer to this outflow as comet 'activity'. The outward diffusion of gas can gradually escape through the comet nucleus' surface, or else remain locked inside of it, until pockets of gas break loose when the gas pressure builds-up and exceeds the strength of the containing walls. In these cases, gas jets out with more vigor. These sort of outbreaks are sometimes even seen at very large distances from the Sun when the comets are no longer supposed to be active.
The escaping gas creates a kind of halo around the comet nucleus, consisting of both gas as well as dust particles of various sizes, which were ejected off the surface along with gas. Later, both the gas and dust interact with Solar radiation. This results in the famous comet 'tails': one gas tail facing away from the Sun, and one dust tail trailing the comet's orbit. These tails could be huge, and although beautiful, they have often triggered reactions of awe and fear by human beings throughout history.
One of the aspects that I have always found fascinating about comets is the diversity of both the nuclei and the composition observed through activity, which might imply that there is more to comets than one would initially guess. Scientists typically think of comets as very primitive. Primitive, as in objects that retain the original composition and structure they had from time of their formation. This belief is prompted by the fact that comets are so small.
Let me clarify what this means. Objects that contain rocky material, and particularly rocky minerals which can incorporate radioactive material, release heat. That is also why the Earth heats up from within. That is why any celestial object containing these radionuclides heats up: planets, moons, asteroids, comets, etc. Radioactive decay releases heat either on short time scales of millions of years, or long time scales of billions of years, depending on which type of radionuclides they harbor.
But wait, aren't comets primarily made of volatile ices? Actually, they are not. And you can read about it here:
Comets are believed to be made primarily from refractory material, and a smaller fraction of volatiles. Therefore, they may, in principle, contain radionuclides which can heat them up. However, having an internal heat source does not guarantee high internal temperatures. This comes from the fact that objects also cool down. Imagine baking a normal-sized potato and a baby-sized potato in your oven. You take them both out of the oven at the same time, which one will cool first to room temperature? Think about poring boiling water in a small glass cup, and in a huge glass bowl. Which one will cool first? Of course the smaller potato and the small glass cup cool first! Mathematically, it is because the cooling time scale goes like the square of size. The smaller the size -- the faster the cooling.
The question of internal heat is therefore a question of competition. It is determined by the competition between the radiogenic heating time scale on the one hand, and the cooling time scale on the other hand. In other words, it's not enough that radionuclides release heat inside the body, because if this heat diffuses out much more rapidly than the rate in which the heat is deposited, the body does not heat at all.
Comets are very small, so their cooling rate is supposed to be much faster than the rate of internal heat deposition. That's why most scientists classically think of them as cold objects that never heated since the time they formed. But that is not what we found in a recent study in collaboration a group of experimental scientists from TU Braunschweig headed by Jürgen Blum. At least, not exactly. While the cooling time scale indeed depends on size, it also depends on other parameters, such as thermal conductivity. This last parameter points to how well your material conducts heat. If it has a very low value, it means that the material is a good insulator, which is another way of saying that the cooling time scale is extremely long. A good example of such a material is glass wool. If you want to make your house green and energy efficient, you line up your walls with this material, which has a low thermal conductivity because it's made from thin stacked layers of glass fibers. It is very porous, and very porous materials are good insulators. It turns out comets can be analogous to that.
Many scientists now think that comets could be made from piles of pebbles. These pebbles are very basic, fundamental structures, that are hypothesized to have formed in the early phase of the formation of the Solar system. They contain a lot of internal porosity. And when piled up, inter-pebble voids constitute as an additional level of porosity. In combination, such materials should have an extremely low thermal conductivity. Based on these new ideas, we have shown that although comets are indeed small, they can still retain more internal heat than previously thought possible. As a consequence, they do heat up past the point where things get interesting. Elevated temperatures can cause highly volatile materials to sublimate and diffuse within the comet nucleus. This highly synergistic study involved an array of new measurements, including producing actual pebbles from micron-sized grains in the lab (movie below shows how a pebble pile looks like). We then used these newly measured relations in order to modify my evolution code for icy bodies SEMIO. It was originally developed for larger icy moons or dwarf planets, but these new modifications made it comet-compatible. Read more about the SEMIO code's capabilities here:
​
​
By performing hundreds of new simulations of the evolution of comet nuclei, given a large grid of various parameters including nuclei sizes, we determined that if comets are indeed composed of pebble piles, they heat enough in order to experience significant internal changes to their composition and structure. We found that for comets whose radii exceed a couple of dozen kilometers, it may be possible to reach temperatures that enable melting of water ice. In this case, liquid water flows rapidly towards the cold surface, freezing before actually reaching it, and leaving behind a volatile-free core. This process is known as rock-water differentiation. An interesting side effect of aqueous flow inside the inner porous refractory core of the comet's nucleus, is that it leads to chemical reactions that alter the rock's composition, release energy and may facilitate the formation of complex organics. There is also a special implication to aqueous flow inside pebble piles, as we have specifically assumed in this study. Laboratory experiments have verified that if pebble piles contain sufficient liquid, they can instantaniously collapse as a result of liquid passing through them and weakening their strength. The following experiment shows this collapse, for pebbles that were produced from a mixture of rock and water ice (credit - Noria Brecher):
​
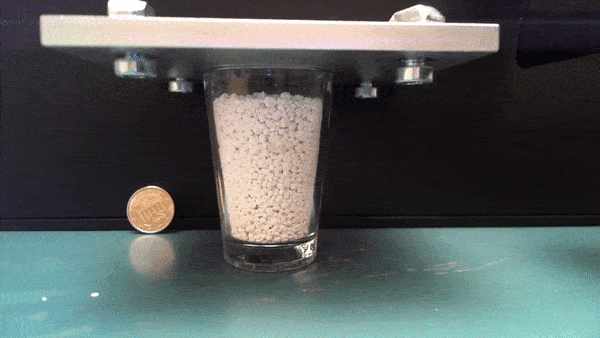
​
​
Our study has given rise to several new hypotheses, one of which is that large comets may actually undergo global collapse due to this process, changing their density and spin state. Another, more general prediction of the model, is that comet nuclei of any size beyond about a kilometer, undergo some kind of internal change. It takes a high temperature to melt water ice, but far lower temperature are required in order to melt high-volatility ices, if they exist in the nucleus, or release hyper-volatile gasses trapped inside other amorphous ices like water or carbon dioxide. Amorphous ices form in space under low pressure conditions, and they have a non-crystalline structure that allows them to trap gasses inside, either when they are co-deposited with the gas, or even later on if gas is streamed through them. When they are heated to about 80-100 K, they start undergoing phase transitions and begin to release the trapped gasses in sequence. Our models predict that this kind of gas release and migration towards the cold surface can start happening even in tiny comet nuclei, due to the low thermal conductivity of pebble piles. And this migration increases with the size of the comet nuclei, until, as previously noted, extremely large comets may even melt water. The internal structure of large comet nuclei is conceptually described in the following schematic.
​
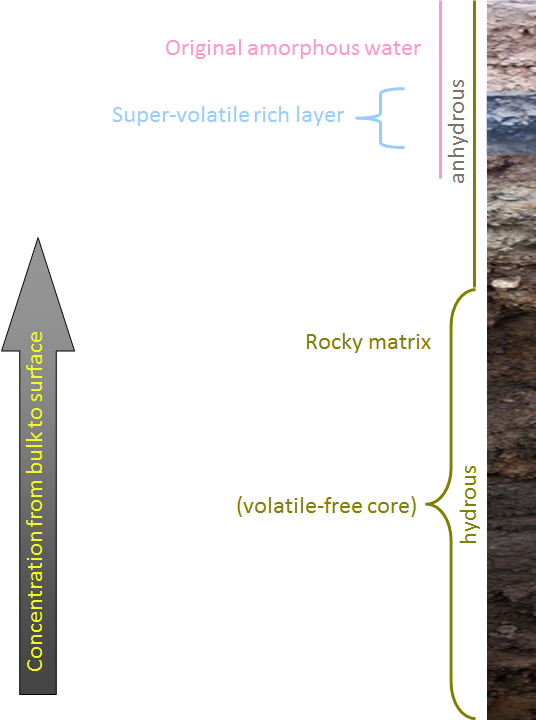
​
​
For much smaller comet nuclei the above schematic is not relevant, however, the model still predicts enrichment of the surface layers through flow of hyper-volatile gasses. The enrichment of the outer layer correlates with the comet's size, because larger comets attain higher temperatures for a bigger portion of their volume. In order to test this prediction, I established a collaboration with a team of comet observers from Scotland, specializing in the analysis of comet composition. It took longer than a year to assemble a proper database, including comet nuclei that have both a reliable size estimate and composition measurements. We carefully circumnavigated various problems that could have spoiled our conclusions. What we found was rather conclusive. For the hyper-volatile carbon monoxide (CO), the correlation between the CO/H2O ratio and the nucleus size was statistically significant to the 3-sigma level. Additionally, we were able to reproduce the observed CO/H2O trend, using our theoretical model, under the assumption that released gasses are re-entrapped in the amorphous ices near the surface (see image below for the observed data points and one example of our various combinations of model realizations, the solid line colors depicting different formation times of the nucleus). This enrichment is is then exhibited in the comet's activity and should be statistically commensurate with the observations. We also find a strong correlation in other volatile gasses, however statistical inference is limited by the relatively small size of our database. We hope that future re-analysis can benefit from more observations.
​
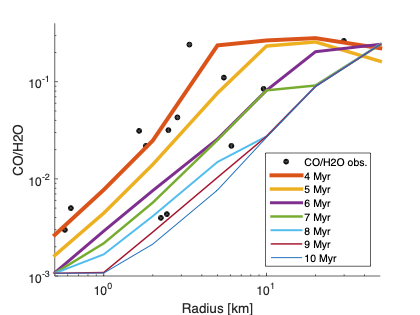