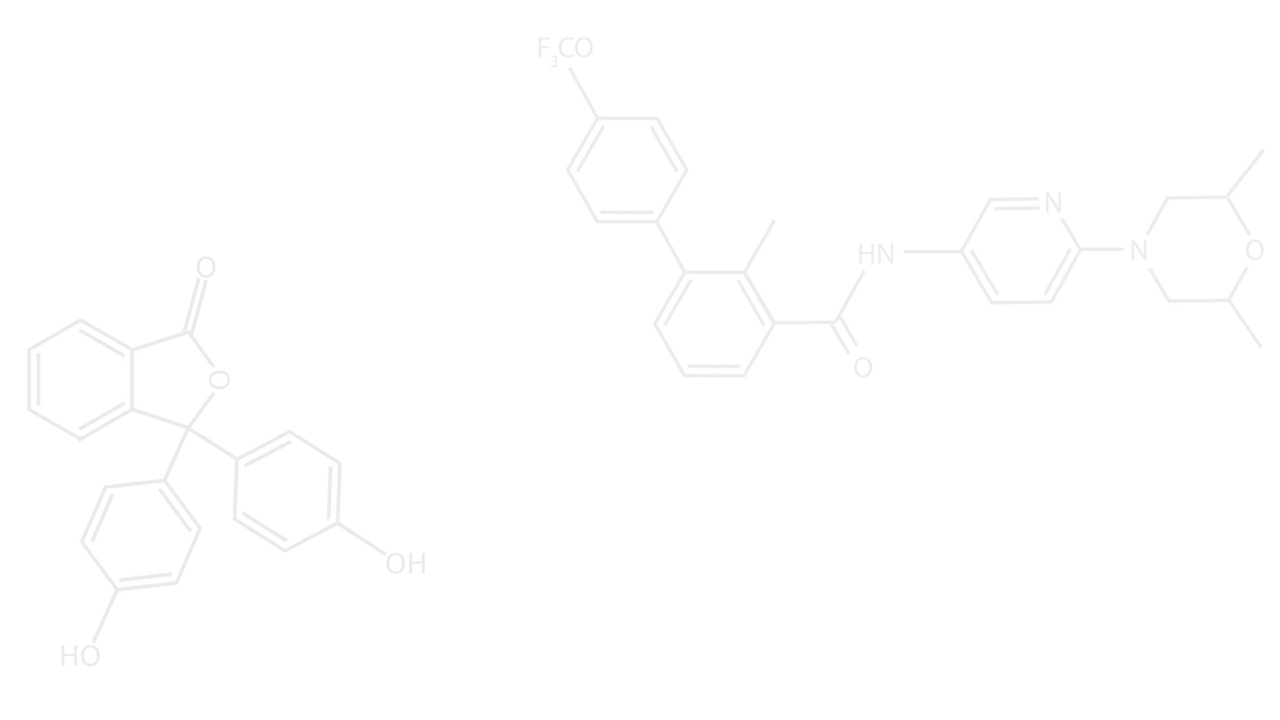
MOONS AND DWARF PLANETS
How do the interiors of satellites and dwarf planets evolve?
Suppose one starts by building a planetary body from a mixture of the available materials in its primordial proto-planetary (if orbiting a star) or proto-satellite (if orbiting a planet) disc. As this disc cools down, the condensed solid materials decouple from the gas and conjoin to form larger planetary objects. Often the available solid materials are silicates (rocks) and volatiles (if these objects form far enough away from the host star, beyond what we call the 'snow line').
​
A planetary object is born. And the simplest premise is to assume it starts off as a homogeneous mixture of all these materials. Here's a list of some of the energy sources available to it, heating the interior over time as the body evolves: radioactive decay of short and long-lived radionuclides, external insolation (irradiation) from the star, tidal heating from a nearby planet or star, phase transitions (such as crystallization of initially amorphous ice), chemical reactions, heat from collisions and some other minor contributions.
​
As heat accumulates, phase transitions occur. For example, consider water melting. Water is lighter than rock, so when it melts one expects a rearrangement of the interior of the planetary body. The lighter, volatile materials, flow toward the surface. If reaching sufficiently cold regions, they freeze again. We end up with a rocky core underlying an icy crust. This process is called differentiation.
During differentiation, liquid water may chemically interact with the rocky material, releasing chemical energy and triggering additional melting. This is a powerful energy source, which also changes the mechanical and thermal properties of the rocks.
​
Typically, scientists tend to assume that differentiation has occurred, however there are very few computer models that can actually track these complex processes. Together with professor Dina Prialnik of Tel-Aviv University, I have developed the SEMIO (structure and evolution modeling of icy objects) computer model which does exactly that. The multi-phase (liquid+gas) flow of water inside a porous solid matrix is tracked, together with the aforementioned energy sources, including that of chemical reactions.
What does a porous matrix mean? Small bodies are often not consolidated pieces of material - instead they have porosity. If you pick up handful of soil from the ground, 20-40% of the volume it would occupy inside your hand would be the empty spaces between interlocking grains. This volume fraction which is not made up of consolidated material, is what we call porosity. We have increasing evidence for many decades that small planetary bodies are porous. Asteroids and comets are known to be porous. Even meteorites that fall onto the Earth from space are often porous. The porosity can be either microscopic or macroscopic or both. Lab experiments show that objects with radii of up to hundreds of kilometers can still have considerable porosity.
​
The complexity of the model comes from self-consistent treatment of all these processes simultaneously. For large bodies, over several hundred kilometers in radius, there are additional complications. The combination of the large pressure from self-gravity and the increasing temperatures can affect their structure, through compaction of the pore space. As porosity diminishes, properties of the mass & heat transport must consistently change. The combination of these thermal, physical, chemical and mechanical processes, and their mutual feedbacks, make this problem solvable strictly via computer simulations.
​
We solve a set of highly coupled, non-linear equations in a numerical relaxation technique. Our model is complex, yet 1-dimensional, and thus appropriate only for global modelling of 3-dimensional objects. Nevertheless, insights based on global modelling may help explain local phenomena, as in the following example.
​
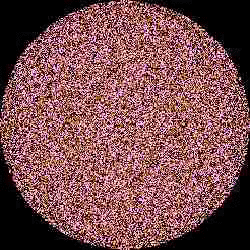
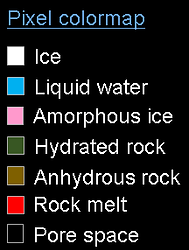
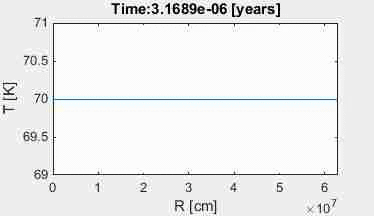
These animations depict the 4.6 Gyr evolution of Charon, the largest satellite of Pluto, since the time of its formation and until the present day. The left frame features the compositional cross-section while the right frame the temperature profile, both as a function of time (which is shown in years at the top of the right frame).
​
As the temperatures rise, differentiation takes place in the following sequence: 1. Crystallization of initially amorphous ice (a thin outer layer remains near the cold surface); 2. melting and migration of water toward the surface while chemically interacting with the rocky core; 3. Dehydration of the inner rocky core, exuding water which accumulates at the base of the icy mantle.
​
Not shown here in these animations, Charon's size undergoes changes as a result of phase changes, compaction and internal redistribution of material. This could lead to the formation of localized surface compression or extension features, when linked with other phenomena such as tidal interaction. In previous work, collaborating with UCLA's Professor Jerry Schubert, we hypothesize that Charon's tectonic belt (highlighted in the below image) could be related to such internal processes.
​
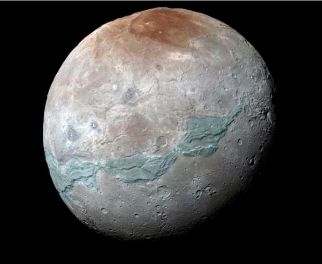
​
For more animations with time dependent boundary conditions see the white dwarf pollution: composition section.
​