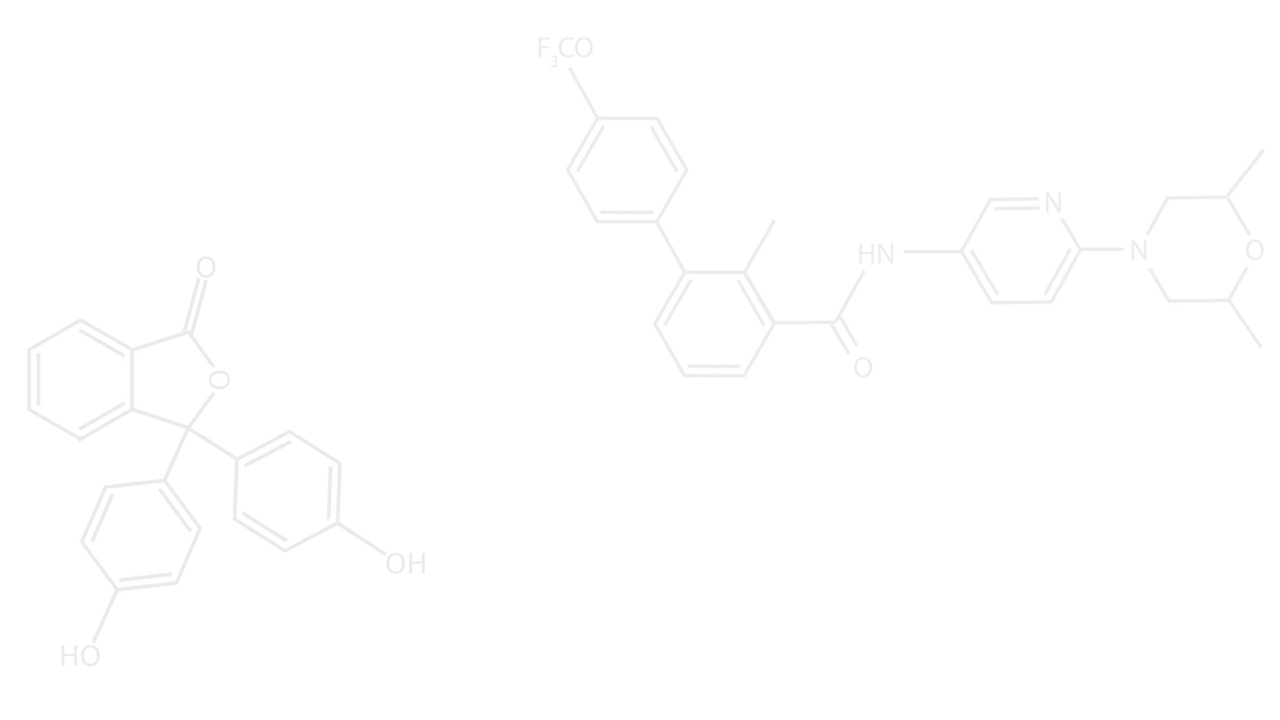
EXO-PLANETARY COMPOSITION
What are planetary systems other than our own Solar system made of? In the last decade or so, we are taking the first steps to answer this question.
​
Thousands of exo-Solar planets have been detected, and sometimes we are able to measure both their mass and size, and therefore, given some error, also their density. By comparing these densities to known Solar system planet densities, we may obtain a crude and inaccurate estimate of what these exo-Solar counterparts are made of. Sometimes it is even possible to take a look at their atmospheres, and find out what the outermost part of their atmosphere is made of. Besides these two methods, we have no idea.
​
Conversely, the observational study of white dwarf stars enables a much more precise way of figuring out the answer to this question. White dwarf stars represent the final evolutionary phase of regular or 'main-sequence' stars like our own Sun. When a main-sequence star starts to run out of fuel, it undergoes a sequence of evolutionary phases in which it expands and thus becomes much more luminous (we thereby call these phases high-luminosity stellar evolution phases). Eventually it sheds its outer shell and the remaining core retains only a fraction of the original star's mass. This core is what we call a white dwarf star. It is very small, on the size scale of a terrestrial planet, and incredibly dense.
​
A lesser known fact is that white dwarf stars also have atmospheres that are dominated by either hydrogen or helium. For many decades, we have known that a large fraction of white dwarf atmospheres (as much as 50%) are also observed to have heavier elements than helium that pollute their atmospheres. These elements, being heavier than helium, are supposed to sink rapidly. Their presence implies that white dwarfs are frequently accreting planetary materials. Occasionally some planetary bodies come close to the white dwarf, tidally disrupt, and eventually create what we call an 'accretion disc'. By observing polluted white dwarf atmospheres, we therefore have the only means to probe the remnants of planetary systems.
​
You can read more about tidal disruptions and disc formation in this link below.
​
​
From the abundances of heavy elements seen in white dwarf atmospheres, we have, for the first time, a really good way of inferring the bulk composition of various planetary systems. Although we do not know what were the exact progenitors of these accretion discs, we typically find that the debris are made up of 'dry' (Earth-like) materials. Only in a handful of cases do we have evidence supporting water-rich progenitors. In our Solar system, objects that lie in the asteroid belt and beyond, are rich in water. This presents a dilemma - if water is so abundant in our Solar system, why not in white dwarf planetary systems?
​
One answer could be related to observational bias, so the statistics might shift in the future. Another could be that water-rich bodies frequently undergo mass loss as a result of sublimation of ice during the high luminosity evolutionary phases of their host stars. This hypothesis however requires testing, since the mass loss rate depends on a great deal of parameters: how massive the host star is, the size of the planetary body, its distance from the star, its structure and composition.
​
For this task I have utilized SEMIO, a sophisticated computer model described in the below link.
​
​
This code was originally developed for Solar system objects, and had to be adapted to work in compatibility with high luminosity host stars that produce extreme and time-variable conditions on the surface of the planetary objects orbiting the star. Time variability originates as the star changes in luminosity and also mass. The latter is important since when the star losses mass it necessarily causes (by conservation of momentum) the orbit of the planetary object to expand, thereby increasing the distance to the star. Luminosity and mass dependencies are obtained from a detailed stellar evolution model called MESA (widely used in the astrophysical community).
The following example shows an animation of the evolution and fate of an object that has a similar size and orbital distance as dwarf planet Ceres, orbiting a Sun-like star as its host. However the evolution exceeds the age of the Solar system (4.6 billion years) and continues until about 12 billion years, encompassing the entire main-sequence phase and then RGB (red giant) and AGB (asymptotic giant) high-luminosity phases of the Sun.
​
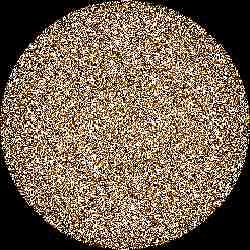
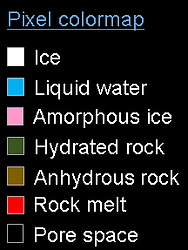
​
This Ceres-like dwarf planet is shown to undergo internal differentiation during the main-sequence phase. During the star's RGB phase the icy mantle slowly sublimates. During the AGB phase the surface temperature is so fervent that even surface rocks get 'baked', forming a dehydrated crust.
By the time of reaching the white dwarf stage, this object ended up being a dry and inert object, lacking in water, with the exception of a thin hydrated (containing H2O) rocky layer near the surface. Models of this kind may perhaps explain the small inferred fraction of water in white dwarfs when observed collectively.
​